mirror of
https://github.com/brl/mutter.git
synced 2024-12-04 22:00:42 -05:00
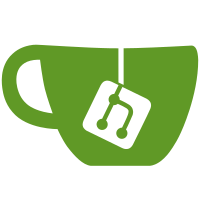
This keeps the existing ClutterBezier implementation but changes the visible API to match the needs of the tablet tool pressure curve: a bezier defined within a [0.0/0.0, 1.0/1.0] box,(sampled into a set of x->y mappings for each possible pressure input x, and a lookup function to get those values out of the curve. This patch moves the internally-only functions to be statics and changes meta_bezier_init() to take only the second and third control point, as normalized doubles. Because internally we still work with integers, the bezier curve now has an integer "precision" that defines how many points between 0.0 and 1.0 we can sample. The meta_bezier_rasterize() function calculates the x->y mapping for each point on the bezier curve given the initial scale of the curve. That value is then available to the caller via meta_bezier_lookup(). Part-of: <https://gitlab.gnome.org/GNOME/mutter/-/merge_requests/3399>
488 lines
13 KiB
C
488 lines
13 KiB
C
/*
|
|
* Authored By Tomas Frydrych <tf@openedhand.com>
|
|
*
|
|
* Copyright (C) 2007 OpenedHand
|
|
*
|
|
* This library is free software; you can redistribute it and/or
|
|
* modify it under the terms of the GNU Lesser General Public
|
|
* License as published by the Free Software Foundation; either
|
|
* version 2 of the License, or (at your option) any later version.
|
|
*
|
|
* This library is distributed in the hope that it will be useful,
|
|
* but WITHOUT ANY WARRANTY; without even the implied warranty of
|
|
* MERCHANTABILITY or FITNESS FOR A PARTICULAR PURPOSE. See the GNU
|
|
* Lesser General Public License for more details.
|
|
*
|
|
* You should have received a copy of the GNU Lesser General Public
|
|
* License along with this library. If not, see <http://www.gnu.org/licenses/>.
|
|
*/
|
|
|
|
#include "config.h"
|
|
|
|
#include <glib.h>
|
|
#include <string.h>
|
|
|
|
#include "meta-bezier.h"
|
|
|
|
/****************************************************************************
|
|
* MetaBezier -- representation of a cubic bezier curve *
|
|
* (private; a building block for the public bspline object) *
|
|
****************************************************************************/
|
|
|
|
/* This is used in meta_bezier_advance to represent the full
|
|
length of the bezier curve. Anything less than that represents a
|
|
fraction of the length */
|
|
#define META_BEZIER_MAX_LENGTH (1 << 18)
|
|
|
|
/*
|
|
* The t parameter of the bezier is from interval <0,1>, so we can use
|
|
* 14.18 format and special multiplication functions that preserve
|
|
* more of the least significant bits but would overflow if the value
|
|
* is > 1
|
|
*/
|
|
#define CBZ_T_Q 18
|
|
#define CBZ_T_ONE (1 << CBZ_T_Q)
|
|
#define CBZ_T_MUL(x,y) ((((x) >> 3) * ((y) >> 3)) >> 12)
|
|
#define CBZ_T_POW2(x) CBZ_T_MUL (x, x)
|
|
#define CBZ_T_POW3(x) CBZ_T_MUL (CBZ_T_POW2 (x), x)
|
|
#define CBZ_T_DIV(x,y) ((((x) << 9) / (y)) << 9)
|
|
|
|
/*
|
|
* Constants for sampling of the bezier
|
|
*/
|
|
#define CBZ_T_SAMPLES 128
|
|
#define CBZ_T_STEP (CBZ_T_ONE / CBZ_T_SAMPLES)
|
|
#define CBZ_L_STEP (CBZ_T_ONE / CBZ_T_SAMPLES)
|
|
|
|
#define FIXED_BITS (32)
|
|
#define FIXED_Q (FIXED_BITS - 16)
|
|
#define FIXED_FROM_INT(x) ((x) << FIXED_Q)
|
|
|
|
typedef gint32 _FixedT;
|
|
|
|
typedef struct _MetaBezierKnot MetaBezierKnot;
|
|
|
|
struct _MetaBezierKnot
|
|
{
|
|
int x;
|
|
int y;
|
|
};
|
|
|
|
/*
|
|
* This is a private type representing a single cubic bezier
|
|
*/
|
|
struct _MetaBezier
|
|
{
|
|
/* The precision, i.e. the number of possible points between 0.0 and 1.0.
|
|
* We require a normalized bezier curve but then later sample to a given
|
|
* precision. The bezier coefficients are scaled up by this factor and later
|
|
* scaled down again during sampling.
|
|
*/
|
|
unsigned int precision;
|
|
|
|
/*
|
|
* bezier coefficients -- these are calculated using multiplication and
|
|
* addition from integer input, so these are also integers
|
|
*/
|
|
int ax;
|
|
int bx;
|
|
int cx;
|
|
int dx;
|
|
|
|
int ay;
|
|
int by;
|
|
int cy;
|
|
int dy;
|
|
|
|
/* length of the bezier */
|
|
unsigned int length;
|
|
|
|
/* the sampled points on the curve */
|
|
double *points;
|
|
};
|
|
|
|
/**
|
|
* meta_bezier_new:
|
|
* @precision: the number of points we can sample between 0.0 and 1.0
|
|
*
|
|
* Create a new bezier curve with the given precision. This precision defines
|
|
* the maximum number of points we can sample and thus thus how much linear
|
|
* interpolation needs to be done when looking up a point on the curve.
|
|
*/
|
|
MetaBezier *
|
|
meta_bezier_new (unsigned int precision)
|
|
{
|
|
MetaBezier *b;
|
|
|
|
g_return_val_if_fail (precision > 0, NULL);
|
|
|
|
b = g_new0 (MetaBezier, 1);
|
|
b->precision = precision;
|
|
b->points = g_new0 (double, precision);
|
|
return b;
|
|
}
|
|
|
|
void
|
|
meta_bezier_free (MetaBezier *b)
|
|
{
|
|
if (G_LIKELY (b))
|
|
{
|
|
g_free (b->points);
|
|
g_free (b);
|
|
}
|
|
}
|
|
|
|
static int
|
|
meta_bezier_t2x (const MetaBezier *b,
|
|
_FixedT t)
|
|
{
|
|
/*
|
|
* NB -- the int coefficients can be at most 8192 for the multiplication
|
|
* to work in this fashion due to the limits of the 14.18 fixed.
|
|
*/
|
|
return ((b->ax * CBZ_T_POW3 (t) + b->bx * CBZ_T_POW2 (t) + b->cx * t) >> CBZ_T_Q)
|
|
+ b->dx;
|
|
}
|
|
|
|
static int
|
|
meta_bezier_t2y (const MetaBezier *b,
|
|
_FixedT t)
|
|
{
|
|
/*
|
|
* NB -- the int coefficients can be at most 8192 for the multiplication
|
|
* to work in this fashion due to the limits of the 14.18 fixed.
|
|
*/
|
|
return ((b->ay * CBZ_T_POW3 (t) + b->by * CBZ_T_POW2 (t) + b->cy * t) >> CBZ_T_Q)
|
|
+ b->dy;
|
|
}
|
|
|
|
/*
|
|
* Advances along the bezier to relative length L and returns the coordinances
|
|
* in knot
|
|
*/
|
|
static void
|
|
meta_bezier_advance (const MetaBezier *b,
|
|
int L,
|
|
MetaBezierKnot *knot)
|
|
{
|
|
_FixedT t = L;
|
|
|
|
knot->x = meta_bezier_t2x (b, t);
|
|
knot->y = meta_bezier_t2y (b, t);
|
|
|
|
#if 0
|
|
g_debug ("advancing to relative pt %f: t %f, {%d,%d}",
|
|
(double) L / (double) CBZ_T_ONE,
|
|
(double) t / (double) CBZ_T_ONE,
|
|
knot->x, knot->y);
|
|
#endif
|
|
}
|
|
|
|
static void
|
|
meta_bezier_sample (MetaBezier *b)
|
|
{
|
|
const size_t N = b->precision;
|
|
const double MIN_EPSILON = 0.00001;
|
|
MetaBezierKnot pos;
|
|
int i;
|
|
double epsilon;
|
|
double t;
|
|
double ts[N]; /* maps pos.x -> t */
|
|
double *points = b->points;
|
|
|
|
for (i = 0; i < N; i++)
|
|
{
|
|
points[i] = -1.0;
|
|
ts[i] = -1.0;
|
|
}
|
|
|
|
ts[0] = 0;
|
|
ts[N - 1] = 1;
|
|
points[0] = 0;
|
|
/* Fill in the last point so linear interpolation (see below) is
|
|
* guaranteed. This should always yield 1.0 anyway. */
|
|
meta_bezier_advance (b, META_BEZIER_MAX_LENGTH, &pos);
|
|
points[N - 1] = pos.y / N;
|
|
|
|
epsilon = 1.0 / N;
|
|
t = epsilon;
|
|
|
|
/* We walk forward from t=0 to t=1.0, calculating every bezier
|
|
* point on the curve and for all x values we remember our matching t.
|
|
* If any x coordinate is missing, we reduce the epsilon and restart
|
|
* with this higher granularity from the last t that gave us a value
|
|
* below the missing x.
|
|
*/
|
|
do
|
|
{
|
|
for (i = 1; i < N; i++)
|
|
{
|
|
if (ts[i] == -1.0)
|
|
{
|
|
t = ts[i - 1];
|
|
epsilon /= 2.0;
|
|
break;
|
|
}
|
|
}
|
|
|
|
while (t < 1.0)
|
|
{
|
|
meta_bezier_advance (b, t * META_BEZIER_MAX_LENGTH, &pos);
|
|
if (pos.x < N)
|
|
{
|
|
if (points[MAX (pos.x - 1, 0)] == -1)
|
|
{
|
|
/* Skipped over at least one x coordinate, let's restart
|
|
* as long as we have have a sensible epsilon. Some curves
|
|
* may never find all points. */
|
|
if (epsilon > MIN_EPSILON)
|
|
break;
|
|
}
|
|
|
|
if (points[pos.x] == -1)
|
|
{
|
|
points[pos.x] = (double)pos.y / N;
|
|
ts[pos.x] = t;
|
|
}
|
|
}
|
|
|
|
t += epsilon;
|
|
}
|
|
}
|
|
while (t < 1.0 && epsilon > MIN_EPSILON);
|
|
|
|
/* Do linear interpolation of the remaining points, if any */
|
|
i = 0;
|
|
while (i < N - 1)
|
|
{
|
|
double *current = &points[++i];
|
|
int prev = points[i - 1];
|
|
|
|
if (*current == -1.0)
|
|
{
|
|
int next_idx = i;
|
|
int next;
|
|
double delta;
|
|
|
|
do
|
|
{
|
|
next = points[++next_idx];
|
|
} while (next == -1.0 && next_idx < N); /* N-1 is guaranteed valid */
|
|
|
|
delta = (next - prev) / (next_idx - i + 1);
|
|
|
|
while (i < next_idx)
|
|
{
|
|
*current = prev + delta;
|
|
prev = *current;
|
|
current++;
|
|
i++;
|
|
}
|
|
}
|
|
}
|
|
|
|
for (i = 0; i < N; i++)
|
|
g_warn_if_fail (points[i] != -1.0);
|
|
}
|
|
|
|
static int
|
|
sqrti (int number)
|
|
{
|
|
#if defined __SSE2__
|
|
/* The GCC built-in with SSE2 (sqrtsd) is up to twice as fast as
|
|
* the pure integer code below. It is also more accurate.
|
|
*/
|
|
return __builtin_sqrt (number);
|
|
#else
|
|
/* This is a fixed point implementation of the Quake III sqrt algorithm,
|
|
* described, for example, at
|
|
* http://www.codemaestro.com/reviews/review00000105.html
|
|
*
|
|
* While the original QIII is extremely fast, the use of floating division
|
|
* and multiplication makes it perform very on arm processors without FPU.
|
|
*
|
|
* The key to successfully replacing the floating point operations with
|
|
* fixed point is in the choice of the fixed point format. The QIII
|
|
* algorithm does not calculate the square root, but its reciprocal ('y'
|
|
* below), which is only at the end turned to the inverse value. In order
|
|
* for the algorithm to produce satisfactory results, the reciprocal value
|
|
* must be represented with sufficient precision; the 16.16 we use
|
|
* elsewhere in clutter is not good enough, and 10.22 is used instead.
|
|
*/
|
|
_FixedT x;
|
|
uint32_t y_1; /* 10.22 fixed point */
|
|
uint32_t f = 0x600000; /* '1.5' as 10.22 fixed */
|
|
|
|
union
|
|
{
|
|
float f;
|
|
uint32_t i;
|
|
} flt, flt2;
|
|
|
|
flt.f = number;
|
|
|
|
x = FIXED_FROM_INT (number) / 2;
|
|
|
|
/* The QIII initial estimate */
|
|
flt.i = 0x5f3759df - ( flt.i >> 1 );
|
|
|
|
/* Now, we convert the float to 10.22 fixed. We exploit the mechanism
|
|
* described at http://www.d6.com/users/checker/pdfs/gdmfp.pdf.
|
|
*
|
|
* We want 22 bit fraction; a single precision float uses 23 bit
|
|
* mantisa, so we only need to add 2^(23-22) (no need for the 1.5
|
|
* multiplier as we are only dealing with positive numbers).
|
|
*
|
|
* Note: we have to use two separate variables here -- for some reason,
|
|
* if we try to use just the flt variable, gcc on ARM optimises the whole
|
|
* addition out, and it all goes pear shape, since without it, the bits
|
|
* in the float will not be correctly aligned.
|
|
*/
|
|
flt2.f = flt.f + 2.0;
|
|
flt2.i &= 0x7FFFFF;
|
|
|
|
/* Now we correct the estimate */
|
|
y_1 = (flt2.i >> 11) * (flt2.i >> 11);
|
|
y_1 = (y_1 >> 8) * (x >> 8);
|
|
|
|
y_1 = f - y_1;
|
|
flt2.i = (flt2.i >> 11) * (y_1 >> 11);
|
|
|
|
/* If the original argument is less than 342, we do another
|
|
* iteration to improve precision (for arguments >= 342, the single
|
|
* iteration produces generally better results).
|
|
*/
|
|
if (x < 171)
|
|
{
|
|
y_1 = (flt2.i >> 11) * (flt2.i >> 11);
|
|
y_1 = (y_1 >> 8) * (x >> 8);
|
|
|
|
y_1 = f - y_1;
|
|
flt2.i = (flt2.i >> 11) * (y_1 >> 11);
|
|
}
|
|
|
|
/* Invert, round and convert from 10.22 to an integer
|
|
* 0x1e3c68 is a magical rounding constant that produces slightly
|
|
* better results than 0x200000.
|
|
*/
|
|
return (number * flt2.i + 0x1e3c68) >> 22;
|
|
#endif
|
|
}
|
|
|
|
void
|
|
meta_bezier_init (MetaBezier *b,
|
|
double x_1,
|
|
double y_1,
|
|
double x_2,
|
|
double y_2)
|
|
{
|
|
double x_0 = 0.0,
|
|
y_0 = 0.0,
|
|
x_3 = 1.0,
|
|
y_3 = 1.0;
|
|
_FixedT t;
|
|
int i;
|
|
int xp = x_0;
|
|
int yp = y_0;
|
|
_FixedT length[CBZ_T_SAMPLES + 1];
|
|
|
|
g_warn_if_fail (x_1 >= 0.0 && x_1 <= 1.0);
|
|
g_warn_if_fail (x_2 >= 0.0 && x_2 <= 1.0);
|
|
g_warn_if_fail (y_1 >= 0.0 && y_1 <= 1.0);
|
|
g_warn_if_fail (y_2 >= 0.0 && y_2 <= 1.0);
|
|
|
|
x_1 *= b->precision;
|
|
y_1 *= b->precision;
|
|
x_2 *= b->precision;
|
|
y_2 *= b->precision;
|
|
x_3 *= b->precision;
|
|
y_3 *= b->precision;
|
|
|
|
#if 0
|
|
g_debug ("Initializing bezier at {{%d,%d},{%d,%d},{%d,%d},{%d,%d}}",
|
|
x0, y0, x1, y1, x2, y2, x3, y3);
|
|
#endif
|
|
|
|
b->dx = x_0;
|
|
b->dy = y_0;
|
|
|
|
b->cx = 3 * (x_1 - x_0);
|
|
b->cy = 3 * (y_1 - y_0);
|
|
|
|
b->bx = 3 * (x_2 - x_1) - b->cx;
|
|
b->by = 3 * (y_2 - y_1) - b->cy;
|
|
|
|
b->ax = x_3 - 3 * x_2 + 3 * x_1 - x_0;
|
|
b->ay = y_3 - 3 * y_2 + 3 * y_1 - y_0;
|
|
|
|
#if 0
|
|
g_debug ("Cooeficients {{%d,%d},{%d,%d},{%d,%d},{%d,%d}}",
|
|
b->ax, b->ay, b->bx, b->by, b->cx, b->cy, b->dx, b->dy);
|
|
#endif
|
|
|
|
/*
|
|
* Because of the way we do the multiplication in bezeir_t2x,y
|
|
* these coefficients need to be at most 0x1fff; this should be the case,
|
|
* I think, but have added this warning to catch any problems -- if it
|
|
* triggers, we need to change those two functions a bit.
|
|
*/
|
|
if (b->ax > 0x1fff || b->bx > 0x1fff || b->cx > 0x1fff)
|
|
g_warning ("Calculated coefficients will result in multiplication "
|
|
"overflow in meta_bezier_t2x and meta_bezier_t2y.");
|
|
|
|
/*
|
|
* Sample the bezier with CBZ_T_SAMPLES and calculate length at
|
|
* each point.
|
|
*
|
|
* We are working with integers here, so we use the fast sqrti function.
|
|
*/
|
|
length[0] = 0;
|
|
|
|
for (t = CBZ_T_STEP, i = 1; i <= CBZ_T_SAMPLES; ++i, t += CBZ_T_STEP)
|
|
{
|
|
int x = meta_bezier_t2x (b, t);
|
|
int y = meta_bezier_t2y (b, t);
|
|
|
|
unsigned int l = sqrti ((y - yp) * (y - yp) + (x - xp) * (x - xp));
|
|
|
|
l += length[i - 1];
|
|
|
|
length[i] = l;
|
|
|
|
xp = x;
|
|
yp = y;
|
|
}
|
|
|
|
b->length = length[CBZ_T_SAMPLES];
|
|
|
|
meta_bezier_sample (b);
|
|
|
|
#if 0
|
|
g_debug ("length %d", b->length);
|
|
#endif
|
|
}
|
|
|
|
/**
|
|
* meta_bezier_lookup:
|
|
* @b: a #MetaBezier curve
|
|
* @pos: the position on the bezier curve in the [0.0, 1.0] range
|
|
*
|
|
* Returns the value of this normalized point on the curve.
|
|
*/
|
|
double
|
|
meta_bezier_lookup (const MetaBezier *b,
|
|
double pos)
|
|
{
|
|
/* The position may be fine-grained than our calculated bezier curve,
|
|
* find the two closest points and do a linear interpolation between
|
|
* those two points.
|
|
*/
|
|
int low_idx = CLAMP ((int)(pos * b->precision), 0, b->precision - 1);
|
|
int high_idx = CLAMP (low_idx + 1, 0, b->precision - 1);
|
|
double low = b->points[low_idx];
|
|
double high = b->points[high_idx];
|
|
double rval = low + (high - low) * (pos - (int)pos);
|
|
|
|
return rval;
|
|
}
|